“You are what you eat” is a phrase popularized by the American nutritionist Victor Lindlahr in the 1930s. Of course, the body’s cells have no way to determine whether the nutrients that reach them come from a four-star gourmet meal or a hot dog on a bun. However, for years, it has been recognized that cancer cells metabolize nutrients in ways that differ from normal cells. Tumor cells frequently sidestep the efficient energy-producing processes of healthy cells, opting instead for metabolic processes that better support aggressive cell proliferation. While this change may support the rapid growth of tumor cells, it also creates potential weaknesses that might be exploited for therapy. If rapidly dividing cancer cells become too reliant on alternative metabolic pathways, therapies that block those pathways could provide a way to disrupt tumor growth.
Can such metabolic treatment approaches be useful in fibrolamellar carcinoma (FLC)? Below is a brief discussion of FLC’s metabolism and “glutamine addiction” as summarized by Mark Furth, Chief Science Officer of FCF.
Beyond the Warburg Effect: Metabolizing for growth
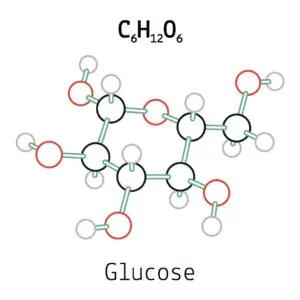
Glucose serves as a major fuel for cells across the biological kingdom. The complete “combustion” of this sugar requires oxygen and depends on specialized organelles called mitochondria which are found in nearly all animal and plant cells. The oxidation process yields carbon dioxide and water, plus a lot of chemical energy which is captured in ATP (adenosine triphosphate) molecules, the universal energy source for living cells. The first stage of that process, glycolysis, occurs in a cell’s cytoplasm and doesn’t require oxygen. In glycolysis, a chain of reactions converts each molecule of glucose into two smaller molecules of pyruvate, plus a small fraction of the ATP that can be obtained from complete oxidation. Typically, the pyruvate formed by glycolysis is then transported into a cell’s mitochondria to undergo oxidation to produce the rest of the cell’s ATP.
A century has passed since the physiologist Otto Warburg observed that cancers consume very large amounts of glucose. However, he was surprised by the way in which tumors metabolize that sugar. In normal healthy organs, nearly all the pyruvate produced by glycolysis moves into the mitochondria for oxidation. Cancer cells, by contrast, channel much of their pyruvate into other biochemical pathways. They also convert a significant portion of it into lactate (often referred to as lactic acid), much of which they “spit out” into the local environment. The abbreviated breakdown of glucose to the 3-carbon products pyruvate and lactate, despite the availability of oxygen, is known formally as “aerobic glycolysis” and is also dubbed “the Warburg Effect”.
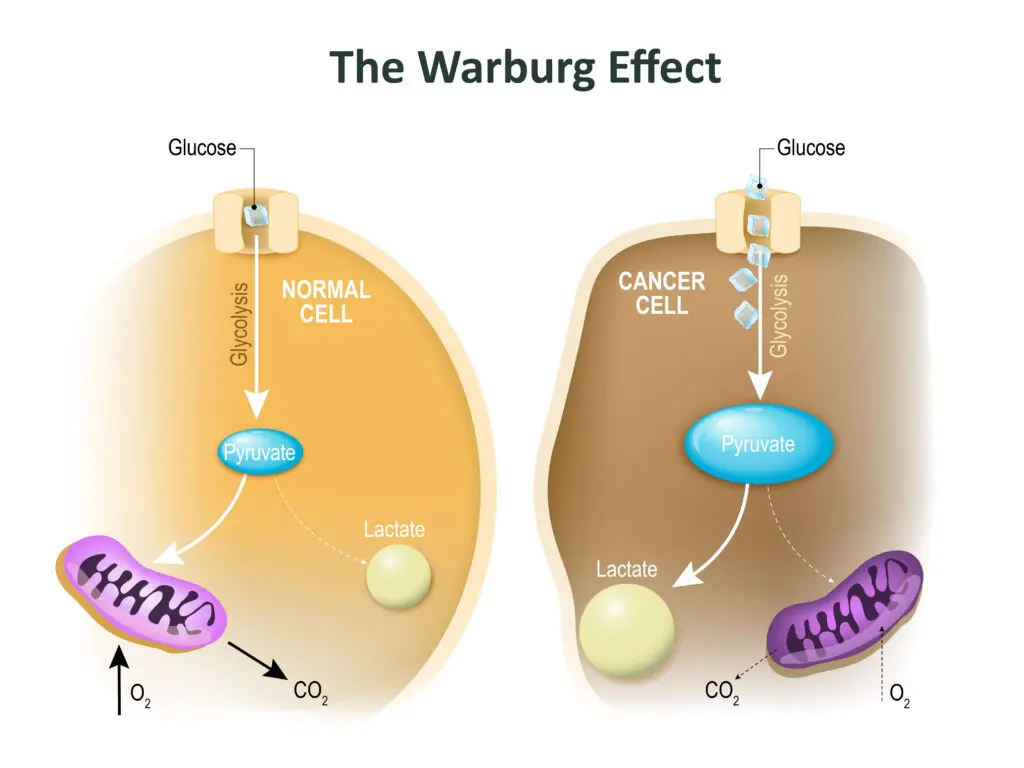
Warburg surmised that aerobic glycolysis resulted from defective mitochondria. He proposed that the failure of mitochondrial function was a fundamental cause of cancer. However, subsequent work showed that most cancers retain the capacity to oxidize glucose. Indeed, experiments indicate that functional mitochondria are essential for cancer cells to divide and form tumors.
Similarly, rapidly dividing non-cancer cells, including those lining the gut, or white blood cells multiplying to fight off an infection, also display high glucose uptake, carry out aerobic glycolysis, and excrete lactate. Work over the past fifteen years has shown that these shared features of cancer cells and expanding normal cell populations reflect situations in which metabolism is “geared toward biomass production and proliferation.” (N.N. Pavlova et al., 2022. Cell Metabolism 34: 355-377). That is, beyond generating sufficient energy, cells preparing to divide must also significantly increase their total content of proteins, RNA and DNA, membranes, and other components. As cancer cells proliferate and spread through the body, they sacrifice maximal production of ATP from glucose to make enough building blocks to support tumor growth.
Glutamine Metabolism
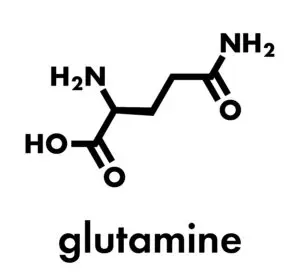
Another nutrient, in addition to glucose, serves as a source of both energy and biomass. Harry Eagle, a pioneer studying cellular metabolism in the 1950s, observed that human cancer cells required remarkably large quantities of glutamine, one of the twenty amino acids that are strung together like beads on a string to make proteins. Subsequent work confirmed that cancers often use significantly more glutamine than would be needed simply to make new protein chains. On the energy side, partial breakdown of glutamine (as for glucose) yields pyruvate and related compounds which can be transported into the mitochondria for oxidation to make ATP. Additionally, compounds derived from glutamine can be used to produce a wide array of cellular components. Notably, glutamine is used to synthesize vital biomolecules containing nitrogen, an element which glucose lacks. These include other amino acids, the precursors needed to build RNA and DNA chains, and a key metabolic regulator called NAD+ (nicotinamide adenine dinucleotide).
Many cancers, including FLC, are addicted to glutamine
When cancer cells over utilize glutamine, a shortfall of this nutrient may not merely slow or arrest their growth. Rather, glutamine deprivation can induce “programmed cell death” (apoptosis). Such a dependency on this amino acid for the survival of cancer cells is known as “glutamine addiction.”
Mark Yarchoan (Johns Hopkins University, JHU) and colleagues recently found evidence that fibrolamellar carcinoma (FLC) is a glutamine addicted cancer (Z. Kamdar et al., 2023. Cancer Research 7 Supp: 3679). As described by Sandy Simon’s lab (Rockefeller University), FLC is associated with mutations that activate protein kinase A (PKA), an enzyme which helps regulate cell metabolism and growth. In most cases, a gene fusion (DNAJB1::PRKACA) creates a chimeric protein designated DP. Work over the past decade has confirmed that DP drives the cancerous growth of FLC cells and is necessary for their survival. The JHU team engineered an existing line of non-FLC mouse liver cancer cells to make the DP protein. The modified cells acquired many of the characteristic features of human FLC.
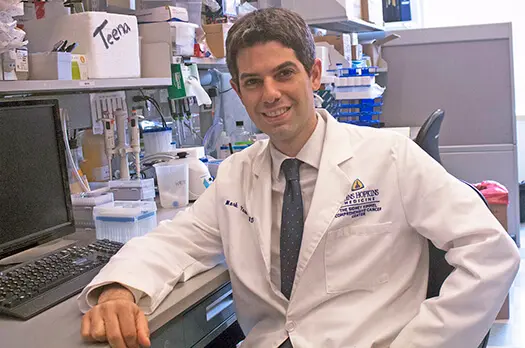
DP induced striking metabolic changes in these engineered FLC-like cells. The modified cells decreased their oxidation of glucose, excreted lactate, and converted lots of glutamine into nitrogen-containing metabolic building blocks. These findings align well with observations of the metabolism of authentic human FLC tumors. Furthermore, the DP-expressing cells died when exposed to a drug that inhibits biochemical reactions involving glutamine.This shows that the DP cancer-driving protein “addicted” the cells to glutamine.
Role of the MYC oncogene in the glutamine addiction of FLC
How does DP promote additional glutamine metabolism? One important element may be the increased expression of MYC, a potent oncogene which has been called “the grand orchestrator of cancer growth” (R. Dhanasekaran et al., 2022. Nat Rev Clin Oncol 19: 23-36). Increased levels of MYC protein are known to reprogram cancer cell metabolism; it channels glutamine into biosynthetic pathways and can cause glutamine addiction.
John Gordan (University of California San Francisco) and colleagues wanted to learn more about how the activation of PKA contributes to turning normal cells into cancer cells. Using human FLC cells, they found that DP increases the amount of MYC protein made from the messenger RNA transcribed from the MYC gene. Such regulation of protein expression is called “translational control.” This may have therapeutic implications, as Gordan’s team found that a drug called Zotatifin, which blocks the increased production of MYC, inhibits the growth of human FLC tumors grafted into special mice (G.K.L. Chan et al., 2023. eLIFE 12: doi 10.7554/eLife.69521).
Drugging glutamine addicted cancers
Efforts to develop drugs that deprive tumors of glutamine began decades ago, soon after the first observations of glutamine addicted cancer cells. A compound isolated from soil bacteria in Peru looked promising. Named 6-diazo-5-oxo-L-norleucine (DON), it can substitute for glutamine in biochemical reactions carried out by metabolic enzymes, inactivating the enzymes and preventing the normal products from being made. The affected pathways include some used to generate energy and others that yield metabolic building blocks. Thus, DON is a broadly acting glutamine antagonist.
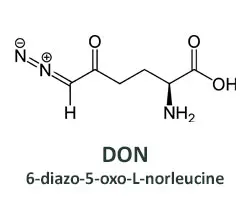
While DON could kill many types of cancer cells, it also caused unacceptable side effects in human patients, especially to the gastrointestinal (GI) tract. The underlying problem was that DON also killed normal intestinal cells that must divide rapidly to maintain the lining of the gut. This made DON a poor candidate for further clinical development.
A team led by Jonathan Powell (Johns Hopkins University) and Barbara Slusher (initially at a pharmaceutical company, now at JHU) looked for a way to modify DON to retain its anti-cancer activity while decreasing its toxicity to normal tissues. They adopted a “wolf in sheep’s clothing” strategy by adding chemical sidechains to DON (see diagram below) that prevent it from binding to the target enzymes and make it inert. The modified compound is called a “prodrug” because once it enters cancer cells, the active form of DON is released by enzymes that “clip off” the sidechains. Because one of these enzymes is much more prevalent in cancer cells than in normal tissues, only an inert compound (“M1 metabolite”) is made in the non-cancerous cells. The selective production of DON from the prodrug in tumors effectively eliminates the dosage limiting GI toxicity, while enhancing the potency against glutamine addicted cancers. Another advantage of the prodrug is that it readily crosses the blood-brain barrier, so it should be effective against brain cancers and metastases.
The Yarchoan group at JHU tested a similar DON prodrug against their engineered FLC-like cells and saw promising anticancer activity. As expected, the drug had little effect on the control non-glutamine addicted cells.
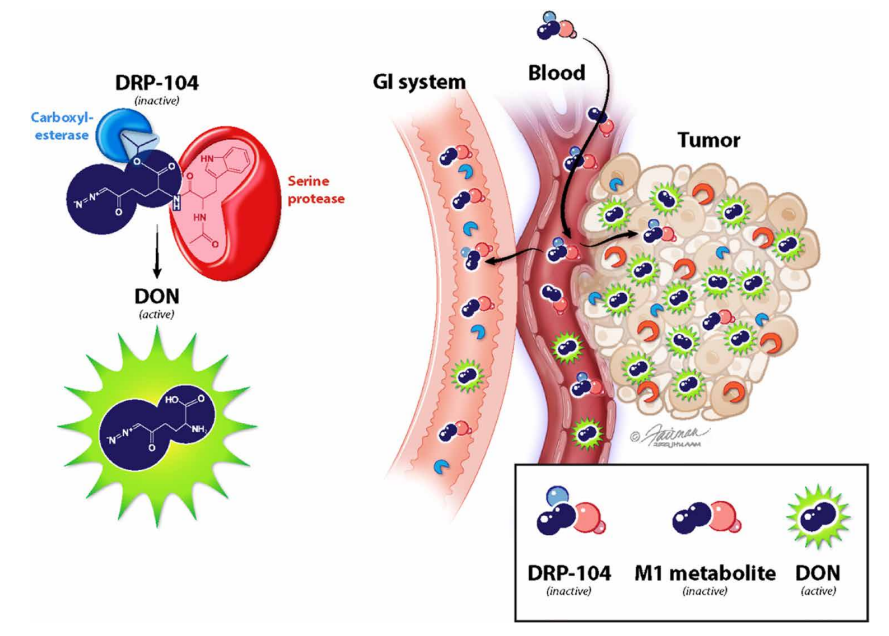
Illustration from Science Advances (Rais et al., Sci. Adv. 8, eabq5925 (2022))
Enhancing immune therapy with a DON prodrug
Beyond altering the energy production and synthesis capacities of cancer cells, metabolic reprogramming strongly impacts the cells’ surroundings — the tumor microenvironment (TME). With the growing success of immunotherapy, it has become apparent that defenses against cancer can be crippled by a suppressive TME. The proliferation of T cells that can recognize and kill cancer cells depends on the same metabolic pathways that the target cancer cells employ. Thus, the hunters and the hunted compete for the same nutrients. Furthermore, cancer cells deplete oxygen and release acids and other toxic metabolites such as ammonia (a particular feature of FLC) into their environment. This creates a TME “that is hostile to anti-tumor immune responses” (R.D. Leone, et al., 2019. Science 366: 1013-1021). In other words, the metabolic reprogramming of cancer cells can inhibit a body’s immune response to a cancer, in addition to directly supporting the growth of the tumor itself.
The JHU team also asked whether the DON prodrug could overcome this “immune evasion” by cancer cells. In mouse models, they found that glutamine blockade both suppressed the cancer cell glutamine metabolism and enhanced the anti-cancer immune response. In addition, they determined that the potential anti-tumor “killer” T cells that infiltrated the mouse tumors were highly proliferative and persisted for longer periods of time after treatment with the DON prodrug. Finally, combining the DON prodrug with an immune checkpoint inhibitor (ICI), which blocks a signal sometimes used by cancer cells to avoid killing by T cells, showed a much stronger anti-tumor response than either drug alone.
Based on these findings, a team led by Drs. Yarchoan and Marina Baretti has begun a clinical trial at JHU that uses a combination of a DON prodrug (DRP-104) and an ICI (durvalumab) to treat FLC patients whose cancer previously progressed on an ICI alone (click here to learn more about the DRP-104 clinical trial). If this approach proves promising in FLC, the investigators anticipate developing a three-component FLC immunotherapy that includes a peptide vaccine (already shown to activate T cells specific to the DP fusion protein), an ICI, and a glutamine blockade.